SALINIDADE E DISPONIBILIDADE DE SUBSTRATOS: IMPLICAÇÕES SOBRE A ATIVIDADE METANOGÊNICA EM DUAS LAGOAS COSTEIRAS DO NORTE FLUMINENSE
Keywords:
acetato, metano, metanogênese, osmorregulação, sulfato.Abstract
Este trabalho teve como objetivo avaliar os efeitos das alterações dos valores de salinidade e da disponibilidade de substratos sobre a metanogênese em duas lagoas costeiras do Norte Fluminense (lagoa Cabiúnas, de água doce à salobra, e lagoa Piripiri, de salobra à hipersalina). Para tanto, realizou-se: (1) levantamento bibliométrico na base Scopus (Elsevier) sobre o tema ‘methanogenesis’ e ‘salinity’; (2) experimentos onde alteramos as concentrações de salinidade e as concentrações de sulfato e acetato. O levantamento bibliométrico demonstrou o aumento das publicações sobre o tema ao longo do tempo, indicando uma nova tendência desse campo de pesquisa no cenário científico. Quanto à adição de NaCl, houve crescimento na produção de metano na lagoa Cabiúnas no aumento de salinidade de 0,9 para 4,0. Na lagoa Piripiri o incremento na produção de metano foi observado com aumento da salinidade de 69,1 para 155,0. Os aumentos maiores da salinidade inibiram a produção de metano na lagoa Cabiúnas e não alteraram na lagoa Piripiri. A adição de sulfato reduziu significativamente a produção de metano na lagoa Cabiúnas, enquanto a adição de acetato a fez aumentar. A adição de sulfato e acetato não alterou a produção de metano na lagoa Piripiri. Estes resultados demonstram o efeito da alteração nos valores de salinidade sobre a produção de metano em ambas as lagoas e que há competição entre a biota metanogênica e a sulfato-redutora na lagoa Cabiúnas enquanto o mesmo não ocorre na lagoa Piripiri.
SALINITY AND SUBSTRATE AVAILABILITY: IMPLICATIONS FOR METHANOGENIC ACTIVITY IN TWO COASTAL LAGOONS IN THE NORTH OF THE STATE OF RIO DE JANEIRO, BRAZIL: This study aimed to evaluate the effects of changes in salinity values and availability of substrates on methanogenesis in two coastal lagoons in Northern Fluminense (Cabiúnas lagoon, from fresh to brackish water, and Piripiri lagoon, from brackish to hypersaline). To this end, it was carried out: (1) a bibliometric survey on the Scopus (Elsevier) base on the theme ‘methanogenesis’ and ‘salinity’; (2) experiments where we changed the salinity concentrations and the sulfate and acetate concentrations. The bibliometric survey showed an increase in publications on the subject over time, indicating a new trend in this field of research in the scientific scenario. As for the addition of NaCl, there was an increase in the production of methane in the Cabiúnas lagoon, with an increase in salinity from 0.9 to 4.0. In the Piripiri lagoon the increment in methane production was observed with an increase in salinity from 69.1 to 155.0. Higher increases in salinity inhibited methane production in the Cabiúnas lagoon and did not change in the Piripiri lagoon. The addition of sulfate reduced while the addition of acetate significantly increased the production of methane in the Cabiúnas lagoon. The addition of sulfate and acetate did not alter the methane production in the Piripiri lagoon. These results demonstrate the effect of the change in salinity values on methane production in both lagoons and that there is competition between methanogenic and sulfate-reducing biota in the Cabiúnas lagoon while the same does not occur in the Piripiri lagoon.References
Achtnich, C., Schuhmann, A., Wind, T., & Conrad, R. 1995a. Role of interspecies H2 transfer to sulfate and ferric iron-reducing bacteria in acetate consumption in anoxic paddy soil. FEMS Microbiology Ecology, 16(1), 61–69. DOI: 10.1016/0168-6496(94)00070-D.
Achtnich C., Bak F. & Conrad R. 1995b. Competition for electron donors among nitrate reducers, ferric iron reducers, sulfate reducers, and methanogens in anoxic paddy soil. Biology and fertility of soils, 19:65–72.
Al-Haj, A. N., & Fulweiler, R. W. 2020. A synthesis of methane emissions from shallow vegetated coastal ecosystems. Global Change Biology, 26(5), 2988–3005. DOI: 10.1111/gcb.15046
Ambühl, H., & Bührer, H. 1975. Zur Technik der Entnahme ungestörter Grossproben von Seesediment: ein verbessertes Bohrlot. Schweizerische Zeitschrift für Hydrologie, 37(1), 175-186. DOI: 10.1007/BF02505185
Baldwin, D. S., & Mitchell, A. 2012. Impact of sulfate pollution on anaerobic biogeochemical cycles in a wetland sediment. Water Research, 46, 965-974. DOI: 10.1016/j.watres.2011.11.065
Bodegom, P. M. Van, & Stams, A. J. M. 1999. Effects of alternative electron acceptors and temperature on methanogenesis in rice paddy soils. Chemosphere, 39(2), 167–182. DOI: 10.1016/S0045-6535(99)00101-0
Burwicz, E. B., Rüpke, L. H., & Wallmann, K. 2011. Estimation of the global amount of submarine gas hydrates formed via microbial methane formation based on numerical reaction-transport modeling and a novel parameterization of Holocene sedimentation. Geochimica et Cosmochimica Acta, 75(16), 4562–4576. DOI: 10.1016/j.gca.2011.05.029
Castro H. F., Williams N. H., & Ogram A. 2000. Phylogeny of sulfate-reducing bacteria. FEMS Microbiology Ecology, 31(1), 1–9. DOI: 10.1111/j.1574-6941.2000.tb00665.x
Chambers, L. G., Reddy, K. R., & Osborne, T. Z. 2011. Short-Term Response of Carbon Cycling to Salinity Pulses in a Freshwater Wetland. Soil Science Society of America Journal, 75(5), 2000–2007. DOI: 10.2136/sssaj2011.0026
Chen, S., Wang, P., Liu, H., Xie, W., Wan, X. S., Kao, S. J., Phelps, T. J., & Zhang, C. 2020. Population dynamics of methanogens and methanotrophs along the salinity gradient in Pearl River Estuary: implications for methane metabolism. Applied Microbiology and Biotechnology, 104(3), 1331–1346. DOI: 10.1007/s00253-019-10221-6
Christian, R. R., & Mazzilli, S. 2007. Defining the coast and sentinel ecosystems for coastal observations of global change. Hydrobiologia, 577(1), 55–70. DOI: 10.1007/s10750-006-0417-4
Connell, J. H. 1978. Diversity in tropical rain forests and coral reefs. Science, 199(4335), 1302-1310. DOI: 10.1126/science.199.4335.1302
Cord-Ruwisch, R., Seitz, H. J., & Conrad, R. 1988. The capacity of hydrogenotrophic anaerobic bacteria to compete for traces of hydrogen depends on the redox potential of the terminal electron acceptor. Archives of Microbiology, 149(4), 350–357. DOI: 10.1007/BF00411655
Dayton, P. K. 1971. Competition, Disturbance, and Community Organization: The Provision and Subsequent Utilization of Space in a Rocky Intertidal Community. Ecological Monographs, 41(4), 351–389. DOI: 10.2307/1948498
Dong, H., Zhang, G., Jiang, H., Yu, B., Chapman, L. R., Lucas, C. R., & Fields, M. W. 2006. Microbial diversity in sediments of saline Qinghai Lake, China: Linking geochemical controls to microbial ecology. Microbial Ecology, 51(1), 65–82. DOI: 10.1007/s00248-005-0228-6
Egger, M., Jilbert, T., Behrends, T., Rivard, C., & Slomp, C. P. 2015. Vivianite is a major sink for phosphorus in methanogenic coastal surface sediments. Geochimica et Cosmochimica Acta, 169, 217–235. DOI: 10.1016/j.gca.2015.09.012
Farjalla, V. F., Faria, B. M., Esteves, F. A., & Bozelli, R. L. 2001. Bacterial density and biomass, and relations with abiotic factors, in 14 coastal lagoons of Rio de Janeiro state. Oecologia Brasiliensis. DOI: 10.4257/oeco.2001.0901.06
Felix, R. W. 2014. Fluxos de metano e dióxido de carbono em lagoas costeiras húmicas: uma abordagem espaço-temporal. Dissertação de Mestrado em Ciências Ambientais e Conservação. Universidade Federal do Rio de Janeiro, Macaé, 2014.
Fonseca, A. L. dos S., Marinho, C. C., & Esteves, F. A. 2019. Acetate and sulphate as regulators of potential methane production in a tropical coastal lagoon. Journal of Soils and Sediments, 19(5), 2604–2612. DOI: 10.1007/s11368-019-02249-y
Hay, M. 1981. The Functional Morphology of Turf-Forming Seaweeds: Persistence in Stressful Marine Habitats. America, 62(3), 739–750.
Ho, A., Mo, Y., Lee, H. J., Sauheitl, L., Jia, Z. & Horn, M. A. 2018. Effect of salt stress on aerobic methane oxidation and associated methanotrophs: a microcosm study of a natural community from a non-saline environment. Soil Biology and Biochemistry, 125:210-214. DOI: 10.1016/j.soilbio.2018.07.013
Jeppesen, E., Beklioğlu, M., Özkan, K., & Akyürek, Z. 2020. Salinization Increase due to Climate Change Will Have Substantial Negative Effects on Inland Waters: A Call for Multifaceted Research at the Local and Global Scale. The Innovation, 1(2), 100030. DOI: 10.1016/j.xinn.2020.100030
Kazumi, J., Caldwell, M. E., Suflita, J. M., Lovley, D. R., & Young, L. Y. 1997. Anaerobic degradation of benzene in diverse anoxic environments. Environmental Science and Technology, 31(3), 813–818. DOI: 10.1021/es960506a
Kuivila, K. M., Murray, J. W., Devol, A. H., & Novelli, P. C. 1989. Methane production, sulfate reduction and competition for substrates in the sediments of Lake Washington. Geochimica et Cosmochimica Acta, 53(2), 409–416. DOI: 10.1016/0016-7037(89)90392-X
Liu, Y., & Boone, D. R. 1991. Effects of salinity on methanogenic decomposition. Bioresource Technology, 35(3), 271–273. DOI: 10.1016/0960-8524(91)90124-3
Lovley, D. R. 1985. Minimum threshold for hydrogen metabolism in methanogenic bacteria. Applied and Environmental Microbiology, 49(6), 1530–1531. DOI: 10.1128/aem.49.6.1530-1531.1985
Lovley, D. R., Dwyer, D. F., & Klug, M. J. 1982. Kinetic analysis of competition between sulfate reducers and methanogens for hydrogen in sediments. Applied and Environmental Microbiology, 43(6), 1373–1379. DOI: 10.1128/aem.43.6.1373-1379.1982
Lyimo, T. J., Pol, A., & Camp, H. J. M. O. Den. 2002. Methane emission, sulphide concentration and redox potential profiles in Mtoni mangrove sediment, Tanzania. Western Indian Ocean Journal of Marine Science, 1, 71–80.
Magenheimer, J. F., Moore, T. R., Chmura, G. L., & Daoust, R. J. 1996. Methane and carbon dioxide flux from a macrotidal salt marsh, Bay of Fundy, New Brunswick. Estuaries, 19(1), 139–145. DOI: 10.2307/1352658
Mahmuduzzaman, M., Ahmed, Z. U., Nuruzzaman, A. K. M., & Ahmed, F. R. S. 2014. Causes of Salinity Intrusion in Coastal Belt of Bangladesh. International Journal of Plant Research, 4(4A), 8–13. DOI: 10.5923/s.plant.201401.02
McLeod, E., Chmura, G. L., Bouillon, S., Salm, R., Björk, M., Duarte, C. M., Lovelock, C. E., Schlesinger, W. H., & Silliman, B. R. 2011. A blueprint for blue carbon: Toward an improved understanding of the role of vegetated coastal habitats in sequestering CO2. Frontiers in Ecology and the Environment, 9(10), 552–560. DOI: 10.1890/110004
Muehe, D., & Valentini, E. 1998. O Litoral do Estado do Rio de Janeiro: uma caracterização físico-ambiental. (Ed.), Rio de Janeiro: FEMAR: p. 123.
Munson, M. A., Nedwell, D. B., & Embley, T. M. 1997. Phylogenetic diversity of Archaea in sediment samples from a coastal salt marsh. Applied and Environmental Microbiology, 63(12), 4729–4733. DOI: 10.1128/aem.63.12.4729-4733.1997
O’Connor, J. J., Fest, B. J., Sievers, M., & Swearer, S. E. 2020. Impacts of land management practices on blue carbon stocks and greenhouse gas fluxes in coastal ecosystems—A meta-analysis. Global Change Biology, 26(3), 1354–1366. DOI: 10.1111/gcb.14946
Odum, W. E. 1988. Comparative ecology of tidal freshwater and salt marshes. Annual Review of Ecology and Systematics, 19, 147–176. DOI: 10.1146/annurev.ecolsys.19.1.147
Oremland, R. S., & Polcin, S. 1982. Methanogenesis and sulfate reduction: competitive and noncompetitive substrates in estuarine sediments. Applied and Environmental Microbiology 44, 1270–1276. DOI: 10.1128/aem.44.6.1270-1276.1982
Oren, A. 1994. The ecology of the extremely halophilic archaea. FEMS Microbiology Reviews, 13(4), 415–439. DOI: 10.1016/0168-6445(94)90063-9
Osudar, R., Klings, K.W., & Bussmann, I. 2017. Effect of salinity on microbial methane oxidation in freshwater and marine environments. Aquatic Microbial Ecology, 80(2), 181-192. DOI: 10.3354/ame01845.
Paine, R. T., & Levin, S. A. 1981. Intertidal landscapes: disturbance and the dynamics of pattern. Ecological monographs, 51(2), 145-178. DOI: 10.2307/2937261
Perski, H. J., Moll, J., & Thauer, R. K. 1981. Sodium dependence of growth and methane formation in Methanobacterium thermoautotrophicum. Archives of Microbiology, 130(4), 319–321. DOI: 10.1007/BF00425947
Petruzzella, A., Marinho, C. C., Sanches, L. F., Minello, M., & Esteves, F. de A. 2013. Magnitude and variability of methane production and concentration in tropical coastal lagoons sediments. Acta Limnologica Brasiliensia, 25(3), 341–351. DOI: 10.1590/s2179-975x2013000300012
Poffenbarger, H. J., Needelman, B. A., & Megonigal, J. P. 2011. Salinity influence on methane emissions from tidal marshes. Wetlands, 31(5), 831–842. DOI: 10.1007/s13157-011-0197-0
Purvaja, R., & Ramesh, R. 2001. Natural and anthropogenic methane emission from coastal wetlands of South India. Environmental Management, 27(4), 547–557. DOI: 10.1007/s002670010169
Reed, D. J. 1995. The response of coastal marshes to sea‐level rise: Survival or submergence? Earth Surface Processes and Landforms, 20(1), 39–48. DOI: 10.1002/esp.3290200105
Sela-Adler, M., Ronen, Z., Herut, B., Antler, G., Vigderovich, H., Eckert, W., & Sivan, O. 2017. Co-existence of methanogenesis and sulfate reduction with common substrates in sulfate-rich estuarine sediments. Frontiers in Microbiology, 8, 1–11. DOI: 10.3389/fmicb.2017.00766
Setubal, R. B., Santangelo, J. M., Rocha, A. D. M., & Bozelli, R. L. 2013. Effects of sandbar openings on the zooplankton community of coastal lagoons with different conservation status. Acta Limnologica Brasiliensia, 25(3), 246-256.
Shiau, Y. J., Lin, C.W., Cai, Y., Jia, Z., Lin, Y. T. & Chiu, C. Y. 2020. Niche differentiation of active methane-oxidizing bacteria in estuarine mangrove forest soils in Taiwan. Microorganisms. 8(8): 1-15. DOI: 10.3390/microorganisms8081248
Šmigáň, P., Rusňák, P., Greksák, M., Zhilina, T. N., & Zavarzin, G. A. 1992. Mode of sodium ion action on methanogenesis and ATPase of the moderate halophilic methanogenesis bacterium Methanohalophilus halophilus. FEBS Letters, 300(2), 193–196. DOI: 10.1016/0014-5793(92)80194-L
Thauer, R. K. 1998. Biochemistry of methanogenesis: a tribute to Marjory Stephenson: 1998 Marjory Stephenson prize lecture. Microbiology, 144(9), 2377-2406. DOI: 10.1099/00221287-144-9-2377
Torres-Alvarado, M. D. R., Fernández, F. J., Ramírez Vives, F., & Varona-Cordero, F. 2013. Dynamics of the methanogenic archaea in tropical estuarine sediments. Archaea, 2013. DOI: 10.1155/2013/582646
Treude, T., Krüger, M., Boetius, A., & Jørgensen, B. B. 2005. Environmental control on anaerobic oxidation of methane in the gassy sediments of Eckernförde Bay (German Baltic). Limnology and Oceanography, 50(6), 1771–1786. DOI: 10.4319/lo.2005.50.6.1771
Vizza, C., West, W. E., Jones, S. E., Hart, J. A., & Lamberti, G. A. 2017. Regulators of coastal wetland methane production and responses to simulated global change. Biogeosciences, 14(2), 431–446. DOI: 10.5194/bg-14-431-2017
Waldron, P. J., Petsch, S. T., Martini, A. M., & Nüslein, K. 2007. Salinity constraints on subsurface archaeal diversity and methanogenesis in sedimentary rock rich in organic matter. Applied and Environmental Microbiology, 73(13), 4171–4179. DOI: 10.1128/AEM.02810-06
Ward, D. M., & Winfrey, M. R. 1985. Interactions between methanogenic and sulfate-reducing bacteria in sediments. Advances in aquatic microbiology, 3, 141-179.
Werner, A. D., & Simmons, C. T. 2009. Impact of sea-level rise on sea water intrusion in coastal aquifers. Ground Water, 47(2), 197–204. DOI: 10.1111/j.1745-6584.2008.00535.x
Weston, N. B., Vile, M. A., Neubauer, S. C., & Velinsky, D. J. 2011. Accelerated microbial organic matter mineralization following salt-water intrusion into tidal freshwater marsh soils. Biogeochemistry, 102(1), 135–151. DOI: 10.1007/s10533-010-9427-4
Williamson, C. E., Saros, J. E., Vincent, W. F., & Smol, J. P. 2009. Lakes and reservoirs as sentinels, integrators, and regulators of climate change. Limnology and Oceanography, 54, 2273-2282. DOI: 10.4319/lo.2009.54.6_part_2.2273
Li, X., Gao, D., & Liu, M. 2019. Composition, diversity and abundance of Candidatus M. oxyfera-like bacteria in response to the estuary salinity gradient. Biogeochemistry, 143(1), 1–14. DOI: 10.1007/s10533-019-00545-w
Xie, W., Zhang, C., Zhou, X., & Wang, P. 2014. Salinity-dominated change in community structure and ecological function of Archaea from the lower Pearl River to coastal South China Sea. Applied Microbiology and Biotechnology, 98(18), 7971–7982. DOI: 10.1007/s00253-014-5838-9
Zhang, G., Bai, J., Tebbe, C. C., Zhao, Q., Jia, J., Wang, W., Wang, X., & Yu, L. 2020. Salinity controls soil microbial community structure and function in coastal estuarine wetlands. Environmental Microbiology, 23(2), 1020–1037. DOI: 10.1111/1462-2920.15281
Zhuang, G. C., Elling, F. J., Nigro, L. M., Samarkin, V., Joye, S. B., Teske, A., & Hinrichs, K. U. 2016. Multiple evidence for methylotrophic methanogenesis as the dominant methanogenic pathway in hypersaline sediments from the Orca Basin, Gulf of Mexico. Geochimica et Cosmochimica Acta, 187, 1–20. DOI: 10.1016/j.gca.2016.05.005
Zhuang, G. C., Heuer, V. B., Lazar, C. S., Goldhammer, T., Wendt, J., Samarkin, V. A., Elvert, M., Teske, A. P., Joye, S. B., & Hinrichs, K. U. 2018. Relative importance of methylotrophic methanogenesis in sediments of the Western Mediterranean Sea. Geochimica et Cosmochimica Acta, 224, 171–186. DOI: 10.1016/j.gca.2017.12.024
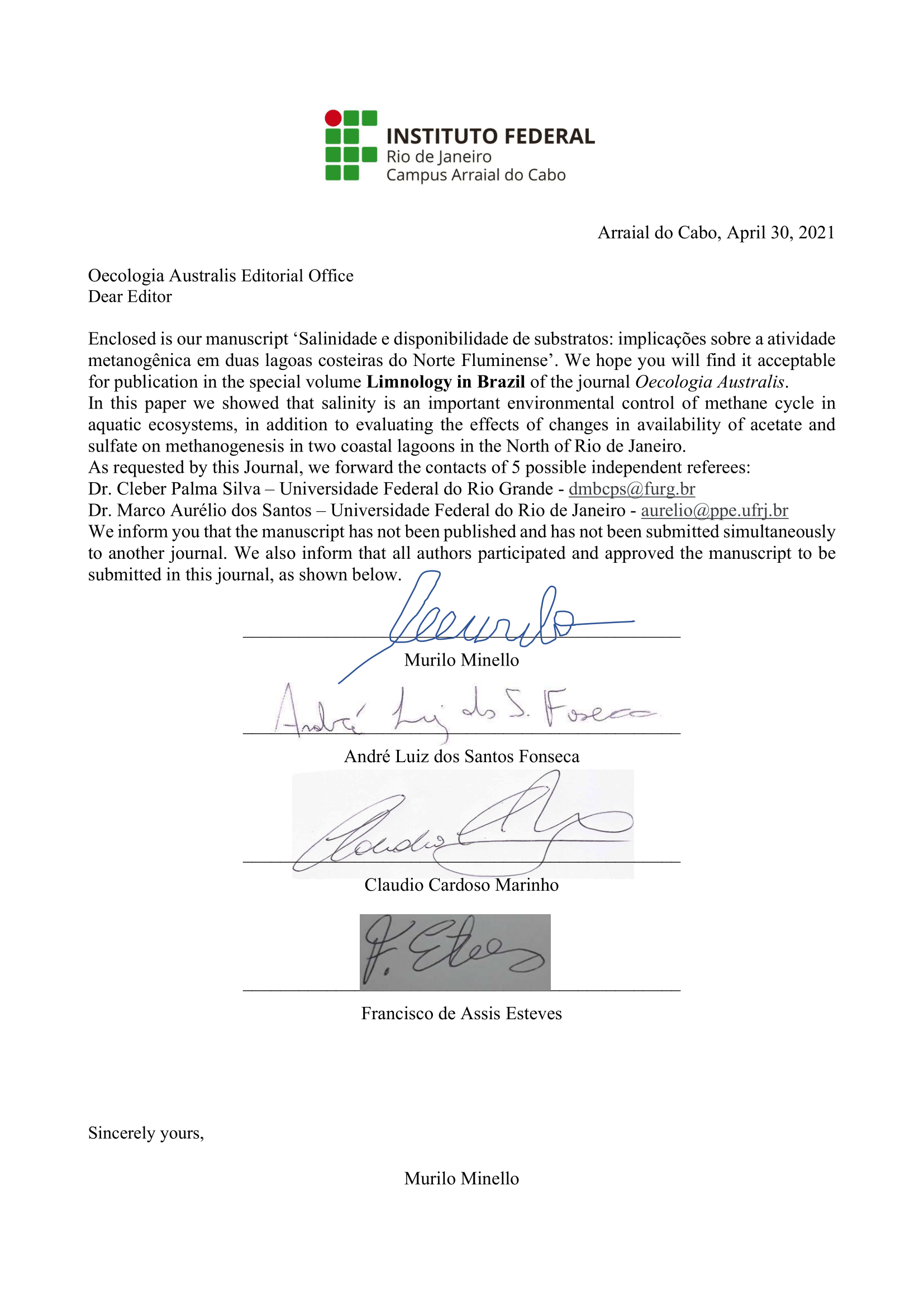